Viewpoint: Studying the cost of decarbonisation
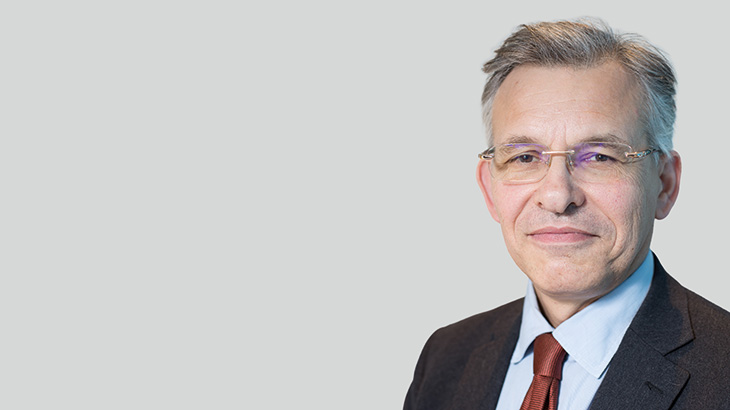
In its 2015 report, Projected Costs of Generating Electricity, the OECD-NEA gave evidence on two key points. First that, in spite of recent high-cost projects - first-of-a-kind in western countries - most new nuclear power plants presented a Levelised Cost Of Electricity (LCOE) comparable with any other generation source, including most Variable Renewable Energy (VRE). LCOE gathers all costs, including Capex and Opex, right up to the connection of a new plant to the grid. Second, the LCOE for VRE did not take into consideration the system costs that the community would be required to pay - grid enhancements to accommodate production far from consumption; balancing due to the low predictability of VRE; and frequency control and electricity backup/storage. The higher the share of VRE in a system, the higher the system cost is.
Aimed at policymakers, the new report presents the main considerations when assessing choices that would effectively achieve deep decarbonisation - lower than 50gCO2/kWh - of the future electricity system. These are:
- What is the most cost-efficient mix to achieve a decarbonisation target with a given share of VRE?
- Which technologies are available, what are their reasonably expected costs and what are their effects on the overall reliability of the electricity system?
- What policies will lead to the long-term investments that deep decarbonisation requires?
Pedagogical approach
The study compares different systems that achieve the same 50gCO2/kWh, which is more or less the agreed target by 2050 to reach the 2-degree climate change scenario. It considers a 'greenfield' approach - defining from scratch the whole system that is able to generate 540 GWh in a year, in order to avoid too many assumptions or disruptions coming from existing technologies or system.
The base case scenario comprises some hydropower, nuclear and some gas. It is the least expensive in LCOE, grid and system costs; it does not have any VRE (their LCOE remains higher than that of nuclear to achieve a given generation in MWh) or VRE dedicated storage, and it has a robust grid and sets a reference for the system costs; it has a carbon price constraint of USD35/tonne CO2 that eliminates any coal generation.
This scenario has some similarities with the French system, which still delivers the cheapest electricity to final consumers - all subsidies and taxes included.
Four scenarios explore an increasing share of VRE in the generation mix - respectively, 10%, 30%, 50% and 75% - and look at the generating capacity needed along with their LCOE plus all additional system costs compared to the base case scenario. In addition to the system costs that were presented in the 2015 report, the new study emphasises the role of the 'profile' or 'utilisation' cost, which reflects the cost of providing residual load when VRE's share increases. The more VRE there is in the system, the more expensive the residual load is.
Not surprisingly, when increasing the share of VRE in generated electricity, the required capacity grows to more than three times the base case. Nuclear decreases significantly and gas capacity more than doubles. The decrease in nuclear is due to the high ramping up/down requirements that VRE imposes on the system. Nuclear can accommodate this but, above a certain level it impacts the load factor to such an extent that nuclear becomes uneconomic. Battery storage has a very limited role to play in any of the study's scenarios. Storage cost is affordable for frequency control or a short-term equilibrium, but in no case can battery storage play a role in medium- to long-term storage because of its cost within the timeframe of the study. Therefore, the balance of electricity when there is no VRE and not enough nuclear has to come from gas.
The cost of electricity - LCOE plus system costs - increases from USD65/MWh in the base case to USD130/MWh when 75% is from VRE. In addition to the LCOE, system costs increase almost exponentially with the share of VRE - rising from a low of USD8/MWh in the 10% VRE scenario to USD50/MWh in the 75% scenario. Grid, balancing and connection costs account for a third and profile costs for two-thirds. Most of the profile costs come from the de-optimisation of the system due to the variability of VRE - because of the correlation in production hours of renewables - which is seen in large existing systems. The more VRE there is deployed, the more expensive the residual load will be and, with a share of VRE higher than 50%, overcapacity when all VRE types are generated leads to more and more curtailment of VRE sources.
The study notes the high volatility of electricity prices in the market. Existing markets are mainly based on the merit order of the marginal cost of generating electricity, but VRE has a marginal cost of zero. This means that when the share of VRE grows to the level of demand capacity, VRE can occasionally meet all the demand and lead to a price of electricity on the market of USD0/MWh along with curtailment of some VRE. From 10% VRE to 75% VRE, the number of hours at a price of USD0/MWh grows from a few hours to almost 4000 per year. In compensation, the number of hours where prices are above USD100/MWh increases. This leads to high volatility in electricity prices and huge price unpredictability.
The report makes five main recommendations:
- recognise and fairly allocate system costs to the technologies that cause them; foster competitive short-term markets for cost-efficient dispatch of available technologies;
- encourage new investment in low carbon technologies by providing stability for investors;
- ensure adequate levels of capacity and flexibility, as well as transmission and distribution infrastructure;
- implement carbon pricing, as the most efficient approach for decarbonising the electricity supply.
- produce suitable policies for the rapid deployment of all available low-carbon technologies in the most cost-effective manner in order to successfully decarbonise the electricity sector
My view
Carbon pricing To set a carbon price seems obvious. The study shows that USD35/tonne CO2 is deemed to be sufficient to eradicate coal from all its scenarios, and not so far from the USD20 that has been achieved in some countries. The sooner this is achieved the better since we all agree that there is an urgent need to decarbonise the energy system.
System costs. Ideally, policies should be developed to make sure that system costs are well analysed and allocated to the source that generates them. In the UK, Dr Dieter Helm proposed the concept of Firm Equivalent Power whereby any VRE source should guarantee its output with some storage it would be responsible for. Practically, in any given system, this would be very difficult to implement.
Benefits from a short-term competitive market. One may question the adequacy of most existing electricity markets: merit order could have been justified in the past when all sources had comparable LCOEs and were fully exposed to the market. With lots of VRE achieving profitability thanks to subsidies, tax credits or power purchase agreements, electricity markets produce prices at zero and are no longer relevant with an increasing share of VRE.
Encouraging new investment in low-carbon technologies. In a market where each and every form of electricity generation is treated according to its own merits - without any subsidies or priority rights - there will be a need for new and clear regulations. With a high share of VRE, existing markets will be very volatile and will present high risks for any long-term investments and their financing. How can policies be designed to attract investment in nuclear power and in non-subsidised VRE?
Capacity, flexibility and infrastructure. There is clear evidence that - apart from hydropower - nuclear, as the only low-carbon dispatchable technology, is essential along with some VRE to achieve a decarbonised electricity system. The cost-effectiveness for the community leads to a balanced system where the value of nuclear and the value of VRE are not both destroyed by having too much VRE. Rather than developing policies with targets for the share of VRE - which will require, in addition, capacity, flexibility and grid infrastructure - would it not be preferable to first set targets in the carbon content and then identify the most cost-efficient electricity system?
When considering the facts about types of technology, their costs including system costs, managing public acceptance of the different technologies and assessing the potential for higher electricity prices, policymakers could create the conditions and market rules for the appropriate pathway.
There are matters that are outside the scope of the NEA study, but which are important for policymakers. One is that, to accommodate a high share of VRE, the system must develop not only transmission and distribution grids, but also incorporate new technologies that do not yet exist to accommodate the fluctuations VRE generation entails. Those costs may have been taken into account in the study, but what about the risks associated with those future technologies? And what about the reliability of such a system and its resilience?
The study did not look at material resources needed in any scenario, but that is a theme to be considered. In essence, VRE has in most areas a limited load factor: to achieve the same generation in GWh, VRE needs three times more capacity than any dispatchable source and would require lots of capacity in storage with, again, a limited load factor. Is this the most efficient way of using the resources the planet can offer?
Another theme for consideration is the acceptability of a given scenario. While existing nuclear energy generation is generally well-accepted, new nuclear may be a challenge; but what about a comparably large deployment of VRE and its footprint? What about the acceptability and feasibility of the distribution/connection requirements?
The NEA's Jan-Horst Keppler said during the webinar given for the study: "An ideal and most cost-effective system should comprise one-third VRE, one-third nuclear power and the remainder hydropower when available, gas with CCS when affordable and flexibility in demand."
My conclusions
Nuclear power will play a key role in future decarbonised systems. While it reliably provides large amounts of dispatchable, low-carbon energy, it faces questions around social acceptance in a few OECD countries. Nevertheless, this study shows how nuclear power still remains the economically optimal choice to satisfy stringent carbon constraints despite the economic challenges for some new generation reactors. Nuclear power's cost advantage lies not in its plant-level costs, though competitive. Instead, it resides in its overall benefits to the electricity system. The plant-level costs of VRE have fallen quite impressively, but its overall costs to the system are not accounted for as its output is clustered during a limited number of high-level hours. All these factors will come into play in the ultimate choices each country makes.
Philippe Costes is senior adviser at World Nuclear Association.
Comments? Please send them to: editor@world-nuclear.org
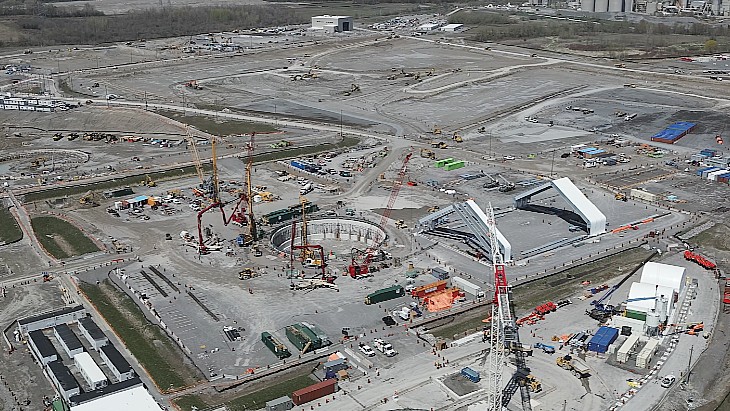
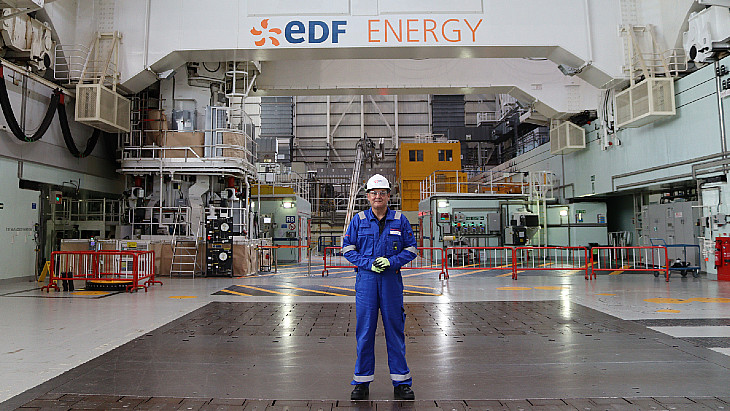
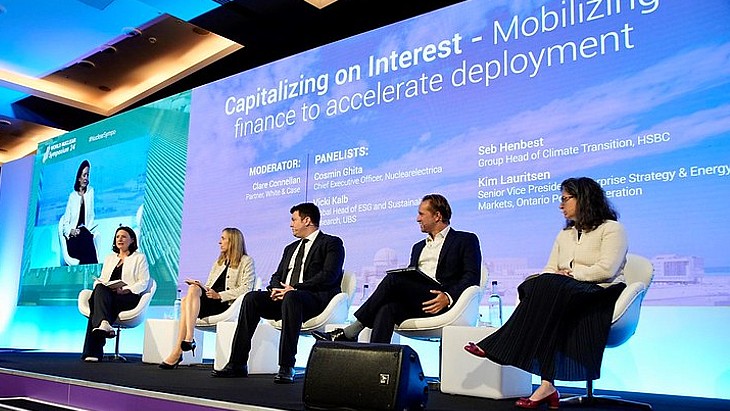
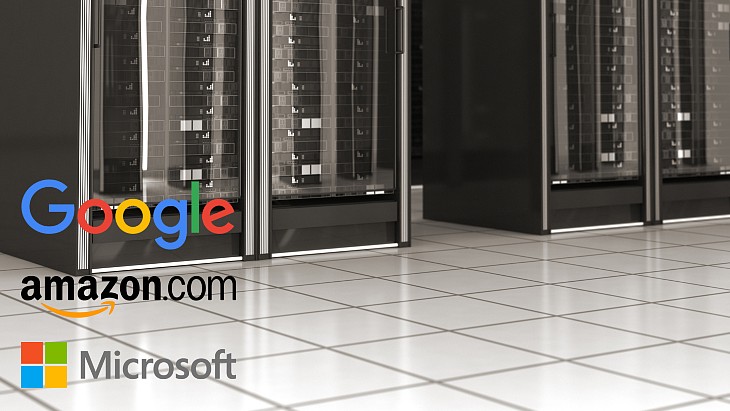
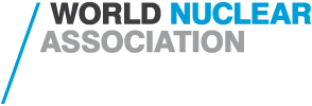