Chemistry, minerals and microbes in nuclear waste management
Current solutions for intermediate and higher activity radioactive wastes storage are temporary. Nuclear waste stores have lifetimes of around 100 years and storage ponds, which dissipate the decay heat from used nuclear fuel and act as powerful radiation shields, several decades. Research into biogeochemical control over radionuclide behaviour is therefore of great value for a deep geological disposal solution, writes Katherine Morris.
The final home of higher activity radioactive wastes is deep geological disposal. This involves encasing the wastes in canisters, burying them hundreds of metres underground in impermeable rock, and backfilling the cavity. Such a project requires a robust safety case looking at the combination of engineering and geology to give a safe "disposal facility".
In my work on radiochemistry, mineralogy and microbiology, I am a strong advocate of interdisciplinary collaboration and finding new scientific pathways. My work scrutinises the destiny of longer-lived, human-made radionuclides as some decay and some integrate back into the environment. In particular, my 'radionuclide biogeochemistry' work focusses on the impact of naturally occurring microbes and minerals on the transport and reactivity of radionuclides and, therefore, the impact of microbial populations on radionuclides.
Key to research into both microbiological and radioactive systems is the challenge of safe handling, transportation and analysis. Some microbial systems can pose biological hazards, whilst high activity, long-lived radionuclides such as technetium, neptunium and plutonium formed in the reactor and uranium in radioactive wastes remain radiotoxic for at least tens of thousands of years.
Through our focus on environmental radioactivity, researchers at The University of Manchester are evolving the contaminated land strategy and raising the profile of radioactive waste research. Fundamental to this is the university's collaboration with Diamond Light Source, the UK's national synchrotron, which is pioneering world-leading and innovative scientific research. At Diamond, particles accelerated to almost the speed of light radiate off energy such as X-rays and gamma-rays, which can be directed at matter to expose underlying properties. We are developing state-of-the-art synchrotron-based analytical methods for radioactive materials.
Synchrotron possibilities
Synchrotron resources offer high energy (2.05-35 keV), secure facilities that expand the scope of quality scientific investigations. One technique, X-ray Absorption Spectroscopy (XAS), which my colleagues and I are employing, probes the bonding and oxidation states of specific elements by exciting core electrons. The high tuneability of synchrotron energy lends itself to switching rapidly between energy ranges for multi-element data collection, and monitoring in situ reactions to the millisecond. The extremely high synchrotron energy provides high resolution data, allowing detection and spatial mapping of chemical elements at extremely low concentrations and in complex heterogeneous samples like wastes, sediments, microbes and minerals. For radioactive research, lower concentration samples mean less radioactivity on the beamline, but as capability to handle samples builds there is a need to push toward 'real world' highly radioactive materials. Building upon previous success, ongoing dialogue has led to a step-change in the capability for analysing radioactive samples at Diamond over the last seven years.
Biogeochemical control over radionuclide behaviour?
In one of our recent experiments on radionuclide biogeochemistry, PhD student Adam Williamson used XAS to detect changes in radionuclide oxidation states in alkaline near-surface sediment samples. These samples were collected at a limeworking site near Buxton, UK and brought to the labs to expose the indigenous, alkaline-tolerant microbe communities to radionuclides. At alkaline pH of 10.5, XAS showed that uranium (VI) and neptunium (V) were substantially bioreduced to less soluble and less mobile uranium (IV) and neptunium (IV), then scavenged onto the natural soil minerals. For the first time, these experiments highlighted the possibility for biogeochemical control over radionuclide behaviour under the high pH conditions some wastes will experience.
In experiments on microbially primed materials, PhD student Diana Brookshaw used XAS on minerals found in igneous rocks, pre-exposed first to the microorganism Geobacter sulfurreducens, then, to radionuclides. In the microbially treated samples, substantial reduction in the mobility of high risk radionuclides occurred because of reaction with Fe(II) produced by microbial reduction of the mineral. XAS allowed the identification of distinct species, including short chains of TcO2, nanocrystalline NpO2 and UO2 materials, and non-crystalline reduced uranium.
These findings help researchers expand our understanding of the interplay between chemistry, minerals and microbes in management and clean-up of nuclear materials. These and other studies suggest that radioactive wastes may develop a 'biobarrier'. This acts as an extra layer of containment for long-lived radionuclides, slowing down their transfer to the biosphere, whilst the waste source supplies microbes with energy. These results can be directly applied to the management and decommissioning of radioactive wastes; and the systems Morris has been exploring could offer biotechnological remediation solutions.
Valuable interdisciplinary research
Research into the biogeochemistry of radionuclides is, unsurprisingly, not a core area of expertise at undergraduate level. Training researchers to work in this exciting environment is key to developing skills to help in environmental clean-up. This interdisciplinary environment is enabled by collaboration of scientists with specialisms in subjects as diverse as microbiology, geochemistry, mineralogy and spectroscopy.
As Marie Curie noted, '...the way of progress is neither swift nor easy', but this is a vibrant research environment, with excellent scientists across undergraduates, postgraduates, post-docs and research staff. The multidisciplinary research capability within the University, wider UK and international community is extraordinary.
Much of this work stemmed from BIGRAD (BIogeochemical Gradient and RADionuclide transport), a large consortium project funded by NERC. Projects like this and its offspring project, EnvRadNet, led by Professor Sam Shaw, Dr Gareth Law and Diamond colleagues, are indispensable. They are forging long-term interdisciplinary and inter-institutional collaborations that boost capability, and widen access to the best facilities and expertise. Cross-disciplinary research not only breaks down barriers between fields, but may be essential to scientific progress.
Katherine Morris
Comments? Please send them to: editor@world-nuclear.org
Professor Katherine Morris is the Dalton Nuclear Institute lead for Nuclear Environment and Waste research at The University of Manchester.
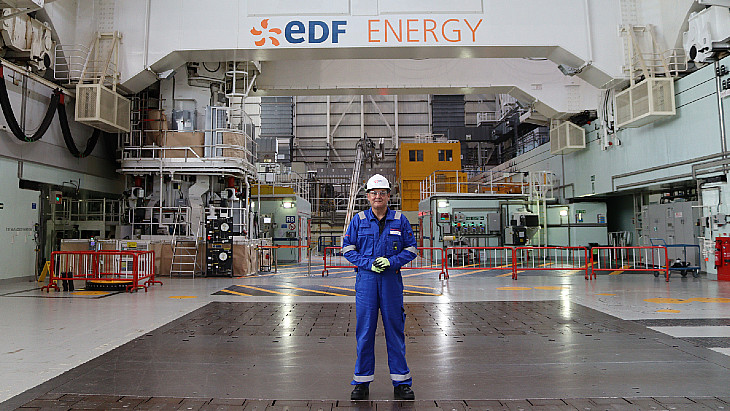
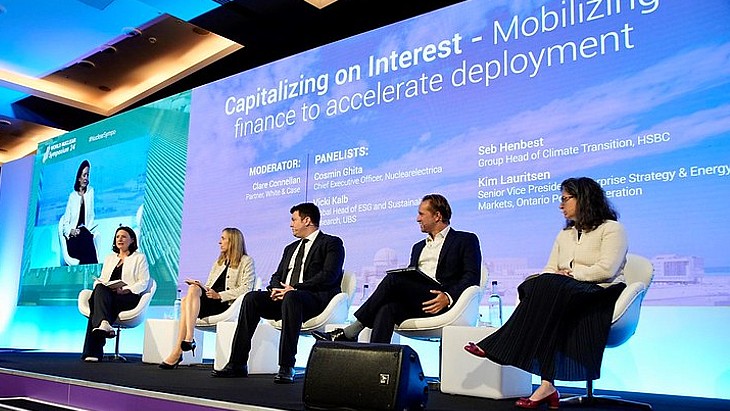
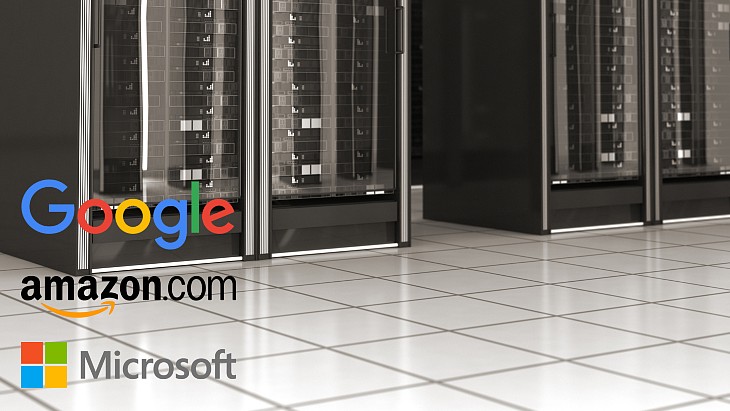
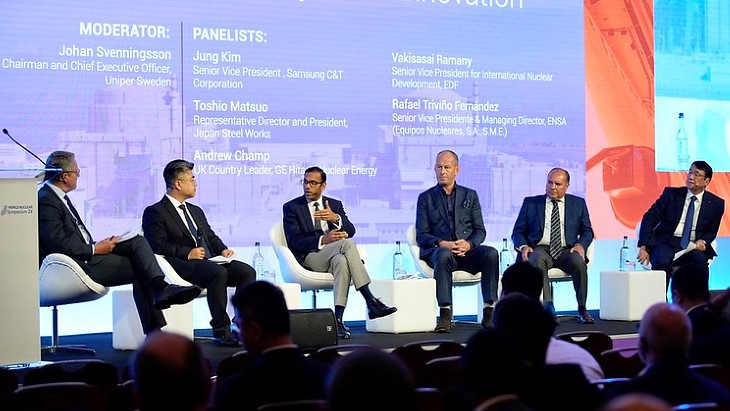
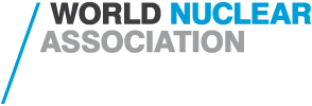